DARPA and US Department of Defense does NOT endorse this post or project idea.
Project Algaepunk 20-77 aims to revolutionize sustainable development by integrating algae cultivation into urban and rural environments. This innovative project proposes the deployment of scalable, modular algae systems on various surfaces to absorb atmospheric carbon, and produce food, fuel, and fiber. By transforming everyday structures into thriving, green landscapes, the project envisions a future where algae play a crucial role in mitigating climate change and promoting resource sustainability.
Algae offer numerous benefits, including high efficiency in carbon capture, rapid growth, and versatility in producing valuable byproducts. Current practices in algae cultivation typically require large land areas or specialized facilities, which limit their broader application. Project Algaepunk 20-77 addresses these limitations by developing a modular system that can be easily installed on buildings, sidewalks, and other surfaces, maximizing the use of existing space without competing with agricultural land.
The project’s primary objectives align with national climate goals and the Department of Defense’s (DoD) climate adaptation strategies. By enhancing the resilience of built and natural infrastructure, Project Algaepunk 20-77 supports efforts to achieve significant greenhouse gas emission reductions and improve energy independence. Furthermore, the project contributes to the DoD’s focus on developing sustainable infrastructure that can withstand and adapt to observed and projected climate changes.
Project Algaepunk 20-77 will be conducted over four years, with rigorous mid-term and final evaluations to ensure the system’s effectiveness and scalability. The project will leverage expertise in engineering, material science, bioengineering, regulatory compliance, and biofuel production to develop and optimize the algae cultivation system. Successful implementation will demonstrate the feasibility of using algae for carbon capture and resource production, setting the stage for widespread adoption and significant environmental impact.
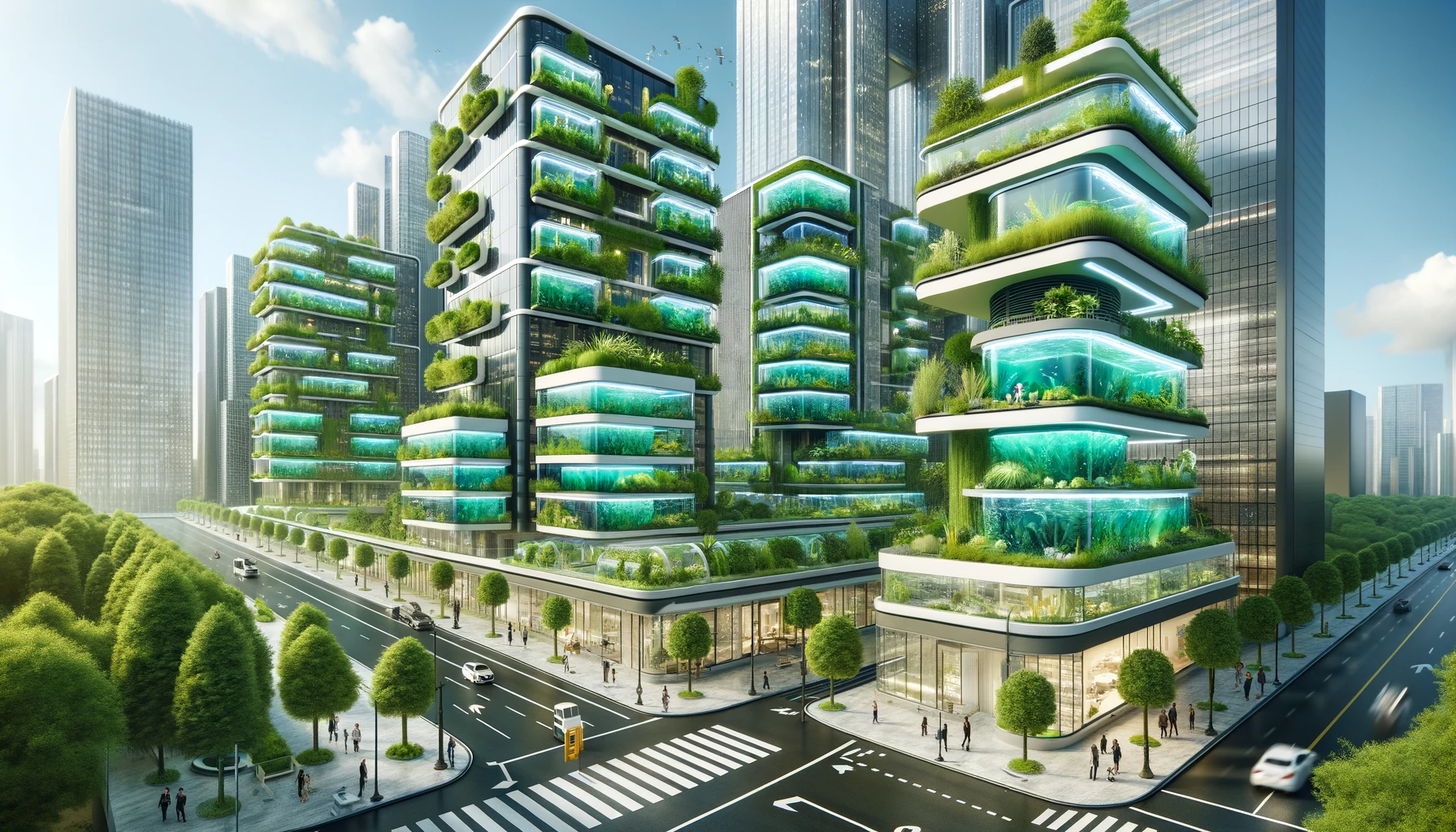
What are you trying to do? Articulate your objectives using absolutely no jargon.
Objective: Develop a low-cost scalable modular system for algae cultivation that can be used on various surfaces to absorb atmospheric carbon, and produce food, fuel, and fiber.
Vision: Transform every sunlit surface into thriving algae cultivation areas, integrating algae into everyday life for a sustainable future.
A modular system for 20 gallons (77 liters) or smaller, bringing the vison of Algaepunk with a 20-77 system.
How Is It Done Today, and What Are the Limits of Current Practice?
Current Practices in Algae Cultivation
Uses of Algae:
- Carbon Capture: Algae is used in carbon capture projects, where it absorbs carbon dioxide from the atmosphere. These projects typically require large expanses of land to cultivate enough algae to make a significant impact.
- Food and Fiber Production: High-value products such as food and fiber are produced in large tanks within extensive facilities. Algae is rich in proteins, vitamins, and minerals, making it a valuable food source. Additionally, its fibers can be used to create sustainable textiles.
- Biofuel Production: Algae is also cultivated for biofuel production, providing an alternative to fossil fuels. This process involves extracting oils from the algae, which can then be converted into biodiesel or other biofuels.
Limitations of Current Practices
Land Use:
- Large Land Areas: Carbon capture projects using algae require vast areas of land, which can be a limiting factor in densely populated or land-scarce regions.
- Competition with Agriculture: Similar to biofuel crops, algae cultivation for carbon capture or fuel can compete with agricultural land. Every acre used for algae cultivation could potentially be an acre lost for food production.
Infrastructure and Cost:
- High Capital Investment: Establishing large-scale algae cultivation facilities, especially for high-value products, requires significant capital investment in tanks, monitoring systems, and processing equipment.
- Operational Costs: Maintaining optimal growth conditions for algae, including temperature, light, and nutrients, involves ongoing operational costs.
Energy Infrastructure:
- Reliance on Rare Earth Minerals: Current sustainable energy solutions, such as photovoltaic solar panels, depend heavily on rare earth minerals. These minerals are often sourced from conflict-affected regions, posing ethical and supply chain challenges.
Scalability and Integration:
- Scalability Issues: Scaling up algae cultivation to meet large-scale demands while maintaining efficiency and sustainability remains a challenge.
- Integration with Existing Land Use: Utilizing existing commercial and residential spaces for algae cultivation is still in its infancy. The integration of algae systems into these areas needs to be optimized for practicality and efficiency.
Environmental Impact:
- Resource Intensive: Algae cultivation can be resource-intensive, requiring significant amounts of water and nutrients, which may not always be sustainable or available in large quantities.
- Waste Management: Managing the byproducts and waste from large-scale algae cultivation poses environmental challenges, including nutrient runoff and disposal of unused biomass.
Current practices in algae cultivation offer valuable solutions for carbon capture, food, fiber, and biofuel production. However, these practices face significant limitations, including competition for land use, high capital and operational costs, reliance on rare earth minerals, scalability challenges, and environmental impacts. Addressing these limitations is crucial for advancing algae cultivation technologies and integrating them effectively into sustainable development frameworks.
Algae is cultivated today for a number of uses including for carbon capture, food, fuel, and fiber. Carbon capture projects that cultivate algae use large areas of land. Higher value products such as food and fiber are grown in large tanks inside large facilities. Growing algae to capture carbon is one solution to address legacy carbon. The issues with cultivating algae is a similar problem with biofuel. With biofuels for every useful agricultural acre used to grow biofuels is potentially an acre lost to produce food. Algae can avoid this issue by using existing land use not currently used for agriculture: commercial, residential.
What is New in the Approach and Why It Will Be Successful?
Innovative Approach: Project Algaepunk 20-77 introduces a novel, low-cost, and scalable modular system for algae cultivation that can be deployed on a variety of surfaces. Unlike traditional large-scale algae farms and indoor facilities, this project aims to transform every sunlit surface—whether commercial, residential, or industrial—into a productive algae cultivation area. The system, designed for 20-gallon (77-liter) modules, leverages the vision of Algaepunk by integrating algae growth into the urban and natural landscape seamlessly.
Key Innovations:
- Modular Design:
- Scalability: The modular system allows for easy scalability. Each 20-gallon unit can be deployed individually or combined to cover larger areas, providing flexibility to adapt to different spaces and needs.
- Ease of Installation: The modular nature simplifies installation and maintenance, making it accessible for widespread adoption in various environments, from rooftops to building facades and open fields.
- Versatile Surface Integration:
- Urban Integration: The system is designed to fit on existing urban infrastructure, such as buildings, sidewalks, and rooftops. This approach maximizes the use of underutilized spaces and reduces the need for additional land, mitigating the competition with agricultural spaces.
- Adaptability: The modular units can be tailored to different architectural designs and environmental conditions, ensuring optimal performance in diverse settings.
- Low-Cost Implementation:
- Materials: Utilizing common and/or recyclable materials like glass and plastic, the system minimizes the need for rare earth minerals. This approach not only reduces costs but also addresses ethical and supply chain concerns associated with traditional renewable energy solutions.
- Simplicity: The design emphasizes simplicity and cost-effectiveness, ensuring that the technology is accessible and affordable for widespread use.
- Multifunctional Output:
- Carbon Capture: The system effectively captures atmospheric carbon, converting it into biomass that can be further processed.
- Food, Fuel, and Fiber Production: The algae cultivated can be used to produce nutritious food, renewable biofuels, and sustainable fibers, addressing multiple needs with a single solution.
- Biochar Production: Converting algae into biochar allows for long-term carbon sequestration and soil improvement, contributing to environmental sustainability.
Reasons for Success:
- Comprehensive Environmental Impact:
- Carbon Sequestration: By capturing and storing atmospheric carbon, the system contributes significantly to reducing greenhouse gas levels, aligning with national and global climate goals.
- Sustainable Resource Use: Utilizing existing surfaces for algae cultivation ensures efficient use of space and resources, promoting sustainability.
- Economic and Social Benefits:
- Job Creation: The widespread adoption of the modular system can stimulate job creation in installation, maintenance, and processing industries.
- Local Production: Producing food, fuel, and fiber locally reduces transportation costs and carbon footprints, fostering community resilience and self-sufficiency.
- Technological and Strategic Advantages:
- Energy Independence: The production of biofuels from algae supports energy independence and security, reducing reliance on fossil fuels and enhancing resilience against supply disruptions.
- Military Applications: The system’s adaptability makes it suitable for military installations, providing on-site production of food, fuel, and other essential resources, thus enhancing logistical capabilities and operational sustainability.
The Algaepunk 20-77 project represents a groundbreaking approach to algae cultivation, combining modular scalability, versatile surface integration, and multifunctional outputs. This innovative system is designed to be low-cost, environmentally sustainable, and socially beneficial, offering a comprehensive solution to contemporary challenges in carbon capture, resource production, and climate change mitigation. Its success is grounded in its ability to integrate seamlessly into urban and natural environments, making it a transformative technology for a sustainable future.
Who Cares? If Successful, What Difference Will It Make?
Project Algaepunk 20-77 offers transformative benefits across multiple sectors, addressing environmental, economic, and national security concerns.
Addressing Climate Goals
National Climate Goals:
- President Biden’s Climate Vision: The project directly supports the goal of reducing U.S. greenhouse gas emissions by 50-52% below 2005 levels by 2030 and achieving 100% carbon pollution-free electricity by 2035. Algae cultivation is a potent tool for carbon capture, as it absorbs carbon dioxide from the atmosphere and can be converted into biochar for long-term carbon storage. This process directly contributes to the nation’s emission reduction targets, enhancing efforts to combat climate change.
Enhancing Military Resilience
Department of Defense (DoD) Climate Adaptation:
- Infrastructure Resilience: The DoD’s Climate Adaptation Plan emphasizes the importance of resilient infrastructure. Integrating algae tanks into military installations can bolster the resilience of both built and natural infrastructure. Algae systems can produce biofuels, reducing dependency on external energy sources and enhancing energy security. This capability is crucial for maintaining operational readiness in diverse and challenging environments.
- Environmental Protection: The project aligns with the DoD’s use of programs like the Readiness and Environmental Protection Integration (REPI) Program and the Sentinel Landscapes Partnership to enhance natural infrastructure. Algae cultivation contributes to integrated natural resource management plans, improving the sustainability and resilience of military installations. This integration helps mitigate environmental impacts and supports long-term ecological health.
Economic and Environmental Impact
Economic Benefits:
- Sustainable Industry Growth: The project fosters the growth of new industries related to algae cultivation, processing, and product development. This growth creates job opportunities and stimulates economic activity, particularly in sectors focused on sustainability and green technology.
- Resource Efficiency: Unlike photovoltaic solar panels, which require rare earth minerals, the algae system primarily uses more abundant materials like glass and plastic, which can be sourced from recycled materials. This reduces reliance on conflict minerals and promotes a more sustainable and ethical supply chain.
Environmental Benefits:
- Carbon Sequestration: Algae cultivation is highly effective for carbon sequestration. By converting algae into biochar, carbon is stored long-term, helping to mitigate global warming and enhance soil health.
- Urban Green Spaces: Algae tanks on buildings, sidewalks, and rooftops create green urban landscapes that improve air quality, reduce urban heat islands, and enhance the aesthetic appeal of cities. This contributes to healthier living environments and promotes public well-being.
Societal Impact
Public Health and Well-Being:
- Improved Air Quality: Algae systems contribute to cleaner air by absorbing carbon dioxide and other pollutants, producing oxygen, and creating healthier urban environments.
- Local Food Production: Algae cultivation for food can provide a local, sustainable source of nutrition, reducing the carbon footprint associated with food transportation and enhancing food security.
What Are the Risks?
Risks to DARPA and the U.S. Military
- Development Challenges: The project involves advanced genetic engineering, biotechnology, and integration with urban infrastructure. There is a risk that the technology may not mature as quickly as needed, leading to delays and increased costs.
- System Reliability: Ensuring the algae cultivation systems are robust and reliable in diverse environmental conditions is challenging. Failures or inconsistencies in system performance could compromise mission-critical applications.
- Security Vulnerabilities: Integrating bioengineered systems into civilian and military installations could introduce new security vulnerabilities. Potential threats include bioterrorism and cyberattacks targeting the control systems of algae tanks.
- Compatibility with Existing Infrastructure: Integrating algae systems with existing military infrastructure requires careful planning and coordination. Misalignment or incompatibility could result in operational disruptions and reduced effectiveness.
- Water Damage Risk: Having large tanks of algae introduces a new risk, that if the tank or tanks were to break it could cause water damage. Many insurances ban or regulate large volumes of liquid because of this risk.
Risks to Society at Large
- Waste Management: The byproducts of algae cultivation, such as unused biomass and wastewater, must be managed sustainably to prevent environmental contamination.
- Market Disruption: The introduction of algae-based products could disrupt existing markets for food, fuel, and textiles. This disruption could negatively impact industries and communities reliant on traditional methods of production.
- Public Acceptance: Gaining public acceptance for widespread algae cultivation in urban areas may be challenging. Concerns over aesthetics, odor, and potential health risks need to be addressed through effective communication and community engagement.
- Genetic Engineering: The use of genetically engineered algae raises ethical questions and regulatory challenges. Escape of a genetically engineered algae could have a negative impact on an ecosystem. Ensuring the safety and compliance of these organisms with existing laws and regulations is critical.
- Human Health Risks: There is a potential risk that exposure to certain algae strains or their byproducts could pose health risks to humans. Thorough testing and safety protocols are necessary to mitigate these risks.
- Operational Safety: Ensuring the safety of personnel involved in the installation, maintenance, and operation of algae systems is essential. Risks include exposure to biohazards, mechanical failures, and chemical handling.
The Algaepunk 20-77 project presents a range of risks that need to be carefully managed to ensure success. Addressing these risks through rigorous planning, robust technology development, and proactive stakeholder engagement is crucial for the project’s success and long-term sustainability.
How much will it cost?
The total estimated cost for Project Algaepunk 20-77 over the four-year period is $46,664,000. This cost includes expenses for equipment, materials, personnel, and travel across the various teams involved in the project.
Team Structure for Project Algaepunk 20-77
Engineering Teams:
- Composition: Three engineering teams
- Responsibilities: Design and develop the modular system for algae cultivation.
- Focus: Ensure the system is scalable, efficient, and adaptable to various surfaces and environments.
Material Science Teams:
- Composition: Two material science teams
- Responsibilities: Support the engineering teams by optimizing the materials used in the construction of the modular units, including algae tanks.
- Focus: Develop durable, cost-effective, and sustainable materials to enhance system performance and longevity.
Bioengineering Team:
- Composition: One bioengineering team
- Responsibilities:
- Initially provide the engineering teams with a wild-type algae strain endemic to the deployment area for the prototype.
- Genetically engineer the algae to optimize for tank cultivation and maximize output.
- Focus: Ensure the algae strains are highly efficient in carbon capture and biomass production.
Regulatory Team:
- Composition: One regulatory team
- Responsibilities:
- Navigate international, federal, state, and local regulations to ensure compliance throughout the project.
- Develop streamlined regulatory frameworks to facilitate the national rollout of the Algaepunk 20-77 modular systems.
- Focus: Address legal and compliance challenges to enable smooth implementation and scalability.
Biofuels Teams:
- Composition: Two biofuels teams
- Responsibilities: Develop a method to convert algae into methanol using pyrolysis, with biochar as a byproduct.
- Focus: Optimize the biofuel production process to ensure it is efficient, cost-effective, and environmentally sustainable.
This structured team approach ensures that each aspect of Project Algaepunk 20-77 is addressed comprehensively, from engineering and material science to bioengineering, regulatory compliance, and biofuel production. Each team will focus on their specialized area, collaborating to achieve the project’s goals of creating scalable, sustainable algae cultivation systems.

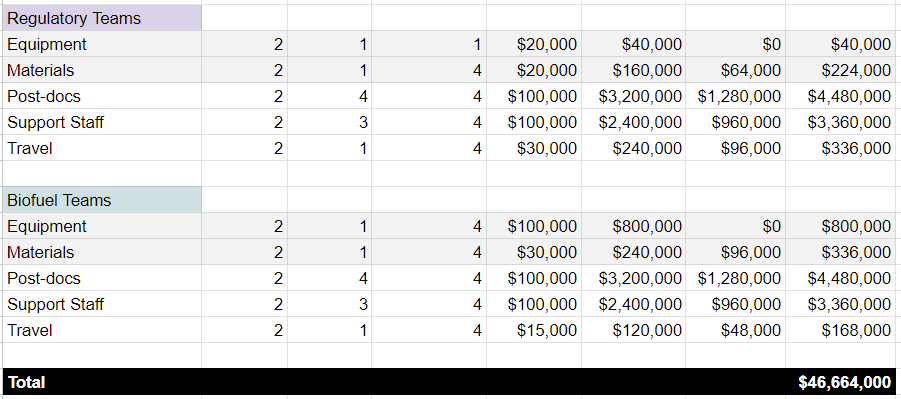
How long will it take?
Project Algaepunk 20-77 is designed to be completed in 4 years. The mid-term exam, conducted after 2 years, will focus on evaluating a single building installation, while the final exam, conducted at the end of the project, will validate the system’s scalability and effectiveness across a larger area, such as a small town or military base. This phased approach ensures comprehensive evaluation and optimization of the algae cultivation systems to achieve project goals.
Project Timeline for Algaepunk 20-77
Duration: 4 years
Year 1: Planning and Initial Development
- Phase 1: Detailed project planning and team formation.
- Phase 2: Initial design and development of the modular algae cultivation system by the engineering teams.
- Phase 3: Selection and initial testing of wild-type algae strains by the bioengineering team.
- Phase 4: Begin optimization of materials by the material science teams.
Year 2: Prototype Testing and Mid-Term Evaluation
- Phase 1: Prototype deployment on a single building, such as a military barrack, to evaluate system performance.
- Phase 2: Collect and analyze data on carbon capture, energy consumption, inputs, and biomass production.
- Phase 3: Mid-term exam to assess scalability, efficiency, and system durability.
- Phase 4: Begin initial regulatory assessments and compliance checks.
Year 3: Optimization and Expansion
- Phase 1: Address any issues identified during mid-term evaluation and optimize the system for larger scale deployment.
- Phase 2: Continue genetic engineering efforts to enhance algae performance in tank cultivation.
- Phase 3: Initiate the development of biofuel production methods, focusing on converting algae biomass into methanol using pyrolysis.
- Phase 4: Conduct further regulatory assessments to facilitate broader deployment.
Year 4: Full-Scale Deployment and Final Evaluation
- Phase 1: Deploy the optimized Algaepunk 20-77 system across a small town or military base.
- Phase 2: Monitor and evaluate overall system performance, including comprehensive data collection on carbon capture, energy use, biomass production, and economic impact.
- Phase 3: Conduct the final exam to validate scalability and long-term sustainability.
- Phase 4: Finalize regulatory framework proposals and prepare for national rollout.
Project Algaepunk 20-77 will be completed within a 4-year timeline. The structured phases ensure comprehensive planning, development, testing, optimization, and large-scale deployment, aligning with the project’s objectives to create a scalable, sustainable algae cultivation system for carbon capture, food, fuel, and fiber production.
What are the mid-term and final “exams” to check for success?
Mid-Term Exam (2 Years)
Objective: Evaluate the performance and feasibility of the Algaepunk 20-77 modular units on a single building within a controlled environment.
Implementation:
- Location: Equip one building with the Algaepunk 20-77 modular units. A military barrack on a base is an ideal candidate due to controlled access and ease of monitoring.
- Metrics to Capture:
- Carbon Capture: Measure the amount of carbon dioxide absorbed by the algae over the testing period.
- Energy Used: Track the energy consumption required to maintain the algae systems, including lighting, temperature control, and water circulation.
- Inputs: Record the inputs needed for algae growth, such as water, nutrients, and any other consumables.
- Dry Weight Biomass: Measure the dry weight of the harvested algae biomass to determine growth efficiency and productivity.
Evaluation Criteria:
- Carbon Sequestration Efficiency: Assess the effectiveness of the system in capturing and sequestering carbon dioxide.
- Energy Efficiency: Analyze the energy consumption relative to the biomass produced and carbon captured.
- Resource Utilization: Evaluate the efficiency of inputs used in relation to the biomass output.
- System Maintenance and Durability: Monitor the ease of maintenance and durability of the modular units under real-world conditions.
Final Exam
Objective: Validate the scalability and overall effectiveness of the Algaepunk 20-77 system by deploying it across an entire small town or military base.
Implementation:
- Location: Equip every building in a small town or a complete military base with the Algaepunk 20-77 modular units.
- Optimized System: Address and resolve any scaling issues identified during the mid-term exam. Implement optimizations for improved performance and efficiency.
Metrics to Capture:
- Overall Carbon Capture: Measure the total carbon dioxide absorbed by the system across all buildings.
- Energy Utilization: Track the total energy consumption for maintaining the system on a larger scale.
- Total Inputs: Record the aggregate inputs required for algae growth across the installation.
- Total Biomass Production: Measure the combined dry weight biomass harvested from all units.
- Economic Impact: Assess the economic benefits, including cost savings on energy, local production of food, fuel, and fiber, and job creation.
- Environmental Impact: Evaluate the broader environmental benefits, such as improvements in air quality and reduction in urban heat islands.
Evaluation Criteria:
- Scalability: Determine the system’s ability to scale up from a single building to an entire community or base without significant loss of efficiency or performance.
- Operational Sustainability: Evaluate the long-term sustainability of the system, including maintenance, resource utilization, and economic viability.
- Community Acceptance: Assess the level of acceptance and satisfaction from the community or base residents regarding the integration of the algae systems.
- Impact on Infrastructure: Analyze the impact of the algae systems on existing infrastructure, including potential benefits and challenges.
The mid-term and final exams for Project Algaepunk 20-77 are designed to systematically evaluate the effectiveness, efficiency, and scalability of the modular algae cultivation systems. By first testing on a single building and then expanding to a small town or military base, these exams will provide comprehensive data on the project’s feasibility and potential for broader implementation. The focus on carbon capture, energy use, resource inputs, and biomass production ensures a thorough assessment of the system’s performance and its contribution to sustainable development and climate resilience
What are the Ethical, Legal, and Societal Impacts of this project?
Ethical Impacts
Environmental Ethics:
- Sustainability: The project promotes sustainability by utilizing algae to capture carbon, produce food, fuel, and fiber, and convert biomass into biochar for long-term carbon storage. This aligns with global efforts to reduce carbon footprints and combat climate change.
- Resource Use: By using common materials like glass and plastic, which can be sourced from recycled materials, the project avoids reliance on rare earth minerals, addressing ethical concerns related to the extraction and supply of these resources.
Health and Safety:
- Public Health: Ensuring that algae cultivation does not pose health risks to humans is crucial. This involves rigorous testing to prevent exposure to harmful algae strains or byproducts.
- Occupational Safety: Safety protocols must be in place for those involved in the installation, maintenance, and operation of the algae systems to protect them from potential biohazards and chemical handling risks.
Legal Impacts
Regulatory Compliance:
- Environmental Regulations: The project must comply with environmental regulations regarding algae cultivation, waste management, and biofuel production. This includes obtaining necessary permits and ensuring that operations do not negatively impact local ecosystems.
- Intellectual Property: Navigating intellectual property rights related to algae cultivation technologies and ensuring fair access to these innovations is essential to prevent legal disputes and promote widespread adoption.
Privacy and Security:
- Data Protection: Managing data related to algae cultivation, such as growth metrics and environmental impact, requires robust data protection measures to prevent misuse and ensure privacy.
- Biosecurity: The use of genetically engineered algae introduces biosecurity concerns. Measures must be taken to prevent the release of genetically modified organisms into the environment, which could have unintended ecological consequences.
Societal Impacts
Economic Impact:
- Job Creation: The widespread adoption of algae cultivation systems can create new job opportunities in installation, maintenance, and processing industries, contributing to economic growth.
- Market Disruption: Introducing algae-based products could disrupt existing markets for food, fuel, and textiles, impacting industries reliant on traditional production methods. Strategies to mitigate these disruptions and support affected industries will be necessary.
Social Equity:
- Accessibility: Ensuring that the benefits of algae cultivation are accessible to all communities, including marginalized and low-income groups, is critical. This includes making the technology affordable and providing training and support for its adoption.
- Community Engagement: Gaining public acceptance for algae cultivation in urban areas involves engaging with communities, addressing concerns about aesthetics, odor, and potential health risks, and demonstrating the environmental and economic benefits.
Global Implications:
- Climate Change Mitigation: By contributing to carbon capture and sustainable resource production, the project supports global climate goals and positions the United States as a leader in innovative climate solutions.
- International Collaboration: The project can foster international collaboration in sustainable technology and climate action, promoting shared knowledge and joint efforts to address global environmental challenges.
Project Algaepunk 20-77 has significant ethical, legal, and societal implications that must be carefully managed to ensure its success and positive impact. Addressing these concerns through robust ethical standards, legal compliance, and proactive community engagement will be crucial for advancing this innovative approach to sustainable development and environmental resilience.